The NICE research project aims
to improve our understanding of the
biophysical effects surrounding
neutron dose deposition in human
tissue.
PI: John
Kildea
Collaborators:
- Michael Evans (McGill)
- Norma Ybarra (McGill)
- Richard Richardson (CNL)
- Jacques Dubeau (Detec Inc.)
- Ioannis Ragoussis (McGill
Genome Centre)
- Patricia Tonin (RI-MUHC
Cancer Research Program)
Current team members:
- Luc Galarneau (research
associate)
- Logan Montgomery, M.Sc.
(Ph.D. candidate)
- Laura Patterson, M.Sc.
(Ph.D. candidate)
- Felix Mathew, M.Sc. (Ph.D.
candidate)
- James Manalad, B.Sc. (M.Sc
candidate)
Former team members:
- Chris Lund (M.Sc.)
- Georges Al Makdessi (M.Sc.)
- Robert Maglieri (M.Sc.)
- Rafael Khatchadourian
(M.Sc.)
|
Part of the NICE
research team with the Nested
Neutron Spectrometer. Left to right:
Robert Maglieri,
Michael Evans, John Kildea, Angel
Licea.
|
Published Research Papers by the
NICE team
F. Mathew, G. Al
Makdessi, L. Montgomery, M. Evans, and J. Kildea.
The
impact of treatment parameter variation on
secondary neutron spectra in high-energy
electron beam radiotherapy. Accepted for publication in the
European Journal of Medical Physics (Physica
Medica), 2020.
F. Mathew, C.
Chilian, L. Montgomery, and J. Kildea. Development
of a passive gold-foil nested neutron
spectrometer to validate the active current-mode
He-3 measurements in a high neutron fluence rate
radiotherapy environment. Nuclear Instruments and Methods
in Physics Research Section A: Accelerators,
Spectrometers, Detectors and Associated
Equipment, 985:164662, 2020.
L. Montgomery, A.
Landry, G. Al Makdessi, F. Mathew, and J.
Kildea. A
novel MLEM stopping criterion for unfolding
neutron fluence spectra in radiation therapy. Nuclear Instruments and Methods
in Physics Research Section A: Accelerators,
Spectrometers, Detectors and Associated
Equipment, 957:163400, 2020.
C. Lund, G. Famulari, L.
Montgomery, and J. Kildea. A
microdosimetric analysis of the interactions of
mono-energetic neutrons with human tissue. Physica Medica, 73:29–42, 2020.
L. Montgomery, M.
Evans, L. Liang, R. Maglieri, and J. Kildea. The
effect of the flattening filter on photoneutron
production at 10 MV in the Varian truebeam
linear accelerator.
Medical physics (doi.org/10.1002/mp.13148),
2018.
F. Ali, J.
Atanackovic, C. Boyer, A. Festarini, J. Kildea,
L. Paterson, R. Rogge, M. Stuart, and R. B.
Richardson. Dosimetric
and microdosimetric analyses for blood exposed
to reactor- derived thermal neutrons. Journal of Radiological
Protection, 2018.
J. Kildea. The
Canadian neutron-induced carcinogenic effects
research program-a research program to
investigate neutron relative biological
effectiveness for carcinogenesis with a
particular focus on secondary (by-product)
neutrons in high-energy radiation therapy. Radiation Environment Medicine,
6(2):55–61, 2017.
R. Maglieri, A.
Licea, M. Evans, J. Seuntjens, and J. Kildea. Measuring
neutron spectra in radiotherapy using the Nested
Neutron Spectrometer.
Medical physics, 42(11):6162–6169, 2015.
Funding: This research program is currently
funded by NSERC
and the Canadian
Space Agency.
Students Rafael Khatchadourian, Robert
Maglieri and Georges Al Makdessi were
supported by grants from the Canadian
Nuclear Safety Commission. The research
of Georges Al Makdessi and Robert Maglieri was
partially supported by MPRTN/CREATE.
Laura Paterson's studies are supported by Canadian Nuclear
Laboratories.
Industrial Partners: We have an
ongoing collaboration with Canadian
Nuclear Laboratories and Detec Inc.
specifically for this project.
Project Description:
The NICE research project aims to
improve our understanding of the biophysical
effects surrounding radiation dose deposition
in human tissue using neutrons.
Ionizing radiation is a potent
carcinogen that is encountered in our natural
and artificial environments. We cannot avoid
it. Thankfully, radiological protection laws
and regulations protect individuals from the
carcinogenic risk that excess ionizing
radiation poses. However, while these measures
guard against unjustified use of radiation,
they cannot protect three distinct
populations:
- Radiotherapy and radiology patients,
for whom the out-of-field radiation dose is
limited only by the justification (i.e.
prescription) of the medical practitioner,
- Astronauts travelling in deep
space who will be exposed to a low-dose
smorgasbord of cosmic rays, and
- Individuals who may be exposed to
unknown radiation levels in the unlikely,
but not impossible, event of a nuclear
accident, atomic bomb, or terrorist attack
involving an improvised nuclear device.
Accordingly, any improvement to our
understanding of radiation-induced
carcinogenesis will help clinicians,
policy-makers, and first responders make
informed decisions to protect these
populations.
In our research program, we are working
to improve our understanding of the etiology
of radiation-induced cancer by studying
neutrons. We are focusing on neutrons because
their effectiveness at causing cancer is known
to be energy-dependent, which suggests an
underlying energy-dependent mechanism by which
neutrons damage DNA. We are examining the
biophysics involved using a combination of
neutron spectral measurements (physics), Monte
Carlo modelling (physics and chemistry), and
cell irradiation experiments (biology).
Specifically, we are aiming to predict the
relative mutation signatures that neutrons of
different energies will induce in the DNA of
irradiated cells. Using the neutron sources
available to us (radiotherapy accelerators,
reactors, and neutron generators), we will
experimentally test our predictions by
irradiating cells in vitro and examining the
mutational signatures induced in them using a
novel single-cell DNA sequencing protocol that
our group is pioneering. Our combination of
modelling and experiment will provide us with
unique insight into the biophysical action of
ionizing radiation.
The results of our research should be of interest to
researchers in the radiation medicine,
space travel, and nuclear emergency
preparedness fields, and our program
will provide a rich multi-disciplinary
environment to train the next generation of
Canadian radiation biology and radiation
protection researchers.
Proton therapy bunker at the Skandion
Clinic, Uppsala, Sweden. Our team measured
the neutron spectrum around one of the
Skandion proton beams in April 2015.
Robert_Maglieri_Thesis.pdf
History
of Neutron Studies at McGill
Our interest in neutrons was originally
motivated by shielding design requirements for
the radiotherapy facilities under construction
at the MUHC Glen site. Graduate student Rafael
Khatchadourian undertook an M.Sc.
project in 2011 supervised by John Kildea
and Michael Evans. The goal of Rafael's work was
to measure neutron ambient equivalent dose in
radiotherapy bunkers and to compare measurements
with Monte Carlo models. Rafael's studies were
funded by a grant from the Canadian
Nuclear Safety Commission.
Following from Rafael's studies, Robert
Maglieri joined our research group for his
M.Sc. research and helped innovate the use of
a new Canadian-made neutron spectrum, the Nested
Neutron Spectrometer (NNS) (Detec Inc.,
Gatineau, Quebec) for use in radiotherapy.
Robert adopted the Maximum-likelihood,
expectation-maximization (MLEM) deconvolution
method to unfold raw NNS data.
Schematic cross section of the
cylindrical NNS system showing the
central He-3 detector and all seven
moderators.
Canadian Common CV
forKildea_John_DGFull_201
The NNS
Our research, which was the subject of
Robert's M.Sc. project, (click
here for the M.Sc. thesis of Robert Maglieri)
demonstrated that the NNS may be used to
measure the energy spectra of neutrons
produced by radiotherapy beams. Our report (Maglieri
et al., 2015,
editor's picks Medical Physics, November
2015) into the first use of this active
neutron detector in the radiation field of a
medical linac has opened the possibility for
practical neutron spectral measurements in
radiotherapy. Until now, such measurements
could only be made using cumbersome, passive
readout techniques requiring days or weeks of
work. With the NNS we can characterize the
neutron spectrum at several points within a
radiotherapy bunker (photon or proton) in just
one afternoon.
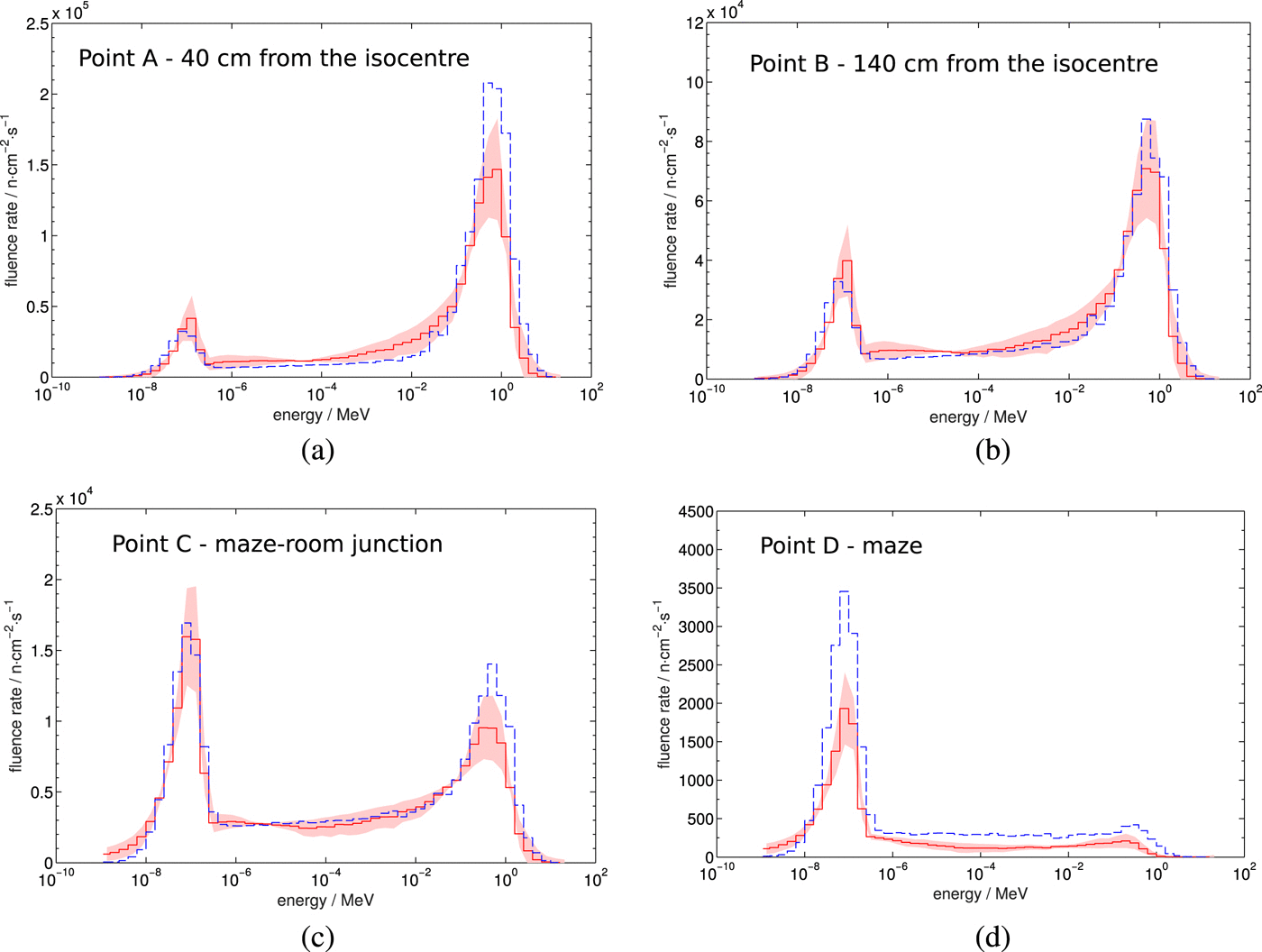
Measured (solid) and simulated (dashed)
neutron spectra in the bunker of a Varian 21EX
linac operated at
18 MV. The shaded region shows the statistical
uncertainty associated with the measurements.
The following papers and presentations
describe our work with the NNS in detail:
From
Neutron Spectral Measurements to Studying
Neutron-induced Carcinogenesis
Our work with the NNS has provided us
with the experience and the motivation to
attempt to convert neutron spectral
measurements into biologically-meaningful dose
estimates. We have shown that we can reliably
measure neutron spectra, but, due to the poor
understanding of neutron-induced
carcinogenesis, we are unable to use these
spectra to advise physicians or patients
regarding biological damage (carcinogenesis).
With this in mind we have formed a
collaboration between McGill, Canadian
Nuclear Laboratories (Chalk River,
Ontario), the Canadian
Nuclear Safety Commission, and Detec Inc.
to investigate neutron carcinogenesis through
a combination of measurements and Monte Carlo
modelling. Our approach is similar to that of
the European ANDANTE
project but it underpinned by our new
measurement technique using the NNS and the
excellent
radio-biological facilities at Chalk
River.
Some members of the McGill-CNL
collaboration on a visit to
CNL's Chalk River laboratories in October,
2015
The problem summarized:
- The relative biological
effectiveness of neutrons for carcinogenesis
is energy dependent. This is borne out in
the ICRP's radiation weighting factors vs
energy plot for neutrons. See below.
- In order to determine neutron
equivalent dose, one must measure the
neutron spectrum. With the NNS, we can
measure neutron spectra!
- But, to convert measured spectra
into biologically meaningful dose (eg
personal equivalent dose), we need reliable
radiation weighting factors. The ICRP
factors are not reliable.
- We aim to study neutron DNA damage
and its relationship to neutron energy as a
way to improve our understanding of neutron
carcinogenesis.
The ICRP radiation
weighting factors for neutrons. Red areas show
approximate
energy ranges for the neutron sources at
CNL and at McGill. Figure adapted from ICRP
Report 103.
Over the next few years we aim to:
- Characterize neutron sources at CNL
and at McGill using the NNS and Monte Carlo
modelling.
- Model (and measure as much as
possible) neutron spectra for realistic
measurement conditions at CNL.
- Select measurement conditions that
provide the most different energies at the
point of measurement (and likely most
different neutron DNA damage for
tissue/cells at that point).
- Irradiate human lymphocyte cells
under the selected measurement conditions
and quantify DNA damage as a function of
neutron energy.
The figure below graphically describes
the objectives of the NICE project. Watch this
space!!
Objectives of the NICE project to investigate
the biophysics underlying neutron DNA damage.
|